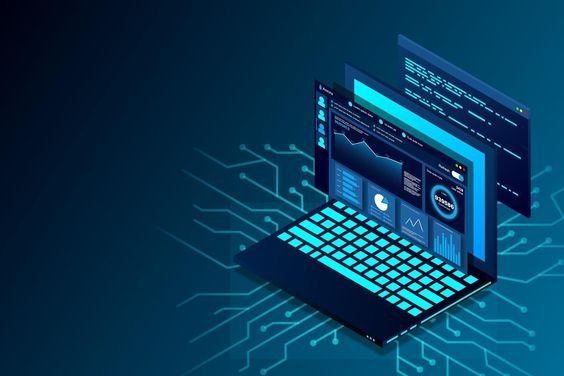
Quantum computing has long captivated scientists, technologists, and futurists alike with its promise of revolutionizing how we solve problems that are currently beyond the reach of classical computers. Despite its potential, quantum computing remains a nascent field. However, recent advances in quantum hardware, software, and algorithms suggest that we are inching closer to realizing its real-world applications. This article delves into the current state of quantum computing, the challenges it faces, and the practical applications that might soon become a reality.
Understanding Quantum Computing: A Brief Overview
To grasp the current progress in quantum computing, it is essential to understand what makes it fundamentally different from classical computing. While classical computers use bits as the smallest unit of information, which can either be in a state of 0 or 1, quantum computers use quantum bits or qubits. Qubits can exist in a state of 0, 1, or both simultaneously, thanks to a property called superposition. Moreover, qubits can become entangled, meaning the state of one qubit can depend on the state of another, even across large distances. These quantum properties enable quantum computers to process information in a massively parallel manner, potentially solving certain problems exponentially faster than classical computers.
The Current State of Quantum Computing
Hardware Advancements
Quantum hardware development is arguably the most challenging aspect of quantum computing. Building a quantum computer requires maintaining qubits in a stable state long enough to perform meaningful calculations, a task complicated by the fragility of quantum states, which are easily disturbed by their environment (a phenomenon known as decoherence).
In recent years, significant progress has been made in quantum hardware. Tech giants like IBM, Google, and Intel, alongside startups such as Rigetti, IonQ, and D-Wave, have been at the forefront of developing quantum processors. In 2019, Google claimed to have achieved “quantum supremacy” with its 54-qubit Sycamore processor, solving a problem in 200 seconds that would have taken a classical supercomputer 10,000 years. While this demonstration was a landmark moment, it was criticized for being a proof-of-concept with limited real-world utility. Nevertheless, it underscored the potential of quantum computing.
As of 2024, quantum processors have grown to accommodate more qubits with improved coherence times and error rates. IBM, for example, unveiled its Eagle processor with 127 qubits in 2021 and plans to release its Condor processor, which will have over 1,000 qubits, by 2025. This scaling up is crucial, as most quantum algorithms require a significant number of qubits to outperform classical computers on practical tasks.
Quantum Software and Algorithms
Developing software and algorithms specifically designed for quantum computers is another area witnessing rapid advancements. Quantum algorithms such as Shor’s algorithm for factoring large numbers and Grover’s algorithm for unstructured search have been known for decades. These algorithms theoretically promise substantial speedups over their classical counterparts.
However, practical applications require more than just these foundational algorithms. Quantum software must also include error correction mechanisms, given the susceptibility of quantum states to errors. Quantum error correction, a field of intense research, aims to mitigate the errors arising from decoherence and other quantum noise sources. Recent progress in this field has been promising, with researchers developing more efficient error correction codes that could significantly extend the practical use of quantum computers.
Furthermore, new quantum algorithms are being developed for various applications, from optimization problems in logistics and finance to material simulation in chemistry and drug discovery. Quantum machine learning, a novel field at the intersection of quantum computing and artificial intelligence, is also emerging as a promising area, leveraging the parallelism of quantum computing to train machine learning models more efficiently.
Challenges Facing Quantum Computing
Despite these advancements, several formidable challenges remain before quantum computers can be deployed for real-world applications.
1. Error Rates and Decoherence
The most significant challenge for quantum computing is maintaining qubit coherence and reducing error rates. Quantum states are highly susceptible to disturbances from their environment, which leads to decoherence. To make quantum computers practically useful, error rates must be reduced to a level where error correction codes can effectively compensate for any errors that occur.
Today’s quantum computers, often described as “noisy intermediate-scale quantum” (NISQ) devices, have relatively high error rates, making them unsuitable for complex calculations without extensive error correction. Research is ongoing to develop qubits with longer coherence times and more robust error correction codes, but it remains a substantial hurdle to achieving practical quantum computing.
2. Scalability
Building a quantum computer that can outperform classical computers on practical tasks requires scaling up the number of qubits significantly. However, as the number of qubits increases, so does the complexity of maintaining their quantum states without errors. Physical limitations, such as cooling requirements (many quantum computers need to be kept at temperatures close to absolute zero) and quantum interference, make scaling up a daunting engineering challenge.
Moreover, connecting qubits in a meaningful way for computation—quantum connectivity—is another issue. While current systems have limited qubit connectivity, research is underway to develop architectures that allow for more efficient entanglement and qubit communication.
3. Quantum Error Correction
Quantum error correction is essential for the practical use of quantum computers, but it remains an area of active research. Current error correction codes require a large number of physical qubits to represent a single logical qubit, making large-scale quantum computing still a distant goal. For instance, some estimates suggest that error correction codes could require thousands of physical qubits to maintain a single logical qubit error-free, highlighting the need for better error correction strategies or more robust qubits.
4. Algorithm and Software Development
While some quantum algorithms, such as Shor’s and Grover’s, have shown theoretical potential, there is still a lack of algorithms tailored to real-world problems that can leverage the power of quantum computers effectively. Developing new algorithms and software frameworks that can run efficiently on quantum hardware is crucial for bridging the gap between theoretical potential and practical application.
5. Infrastructure and Cost
Building and maintaining quantum computers is expensive. The infrastructure required—ranging from cryogenic cooling systems to error correction mechanisms—adds to the cost and complexity. Moreover, developing a quantum computing ecosystem that includes hardware, software, and skilled personnel requires significant investment.
Real-World Applications: How Close Are We?
Despite the challenges, quantum computing is gradually moving from theoretical research to practical applications. Several areas are particularly promising for near-term quantum applications:
1. Optimization Problems
Many industries, such as logistics, finance, and energy, involve complex optimization problems where the goal is to find the best solution among many possible ones. Quantum computers, due to their ability to explore multiple solutions simultaneously, hold promise for solving these problems more efficiently. Companies like Volkswagen and BMW are already exploring quantum computing for optimizing traffic flow and supply chain management, respectively.
2. Drug Discovery and Material Science
Quantum computers can simulate molecular structures and interactions at a quantum level, a task that classical computers find extremely challenging. This capability could revolutionize drug discovery by enabling researchers to model and analyze complex molecules more accurately, potentially leading to the discovery of new drugs and materials. Companies like IBM and startups like ProteinQure and Qubit Pharmaceuticals are pioneering research in this area.
3. Cryptography
Quantum computing poses both a threat and an opportunity for cryptography. Shor’s algorithm, for example, could theoretically break widely used encryption methods like RSA, which relies on the difficulty of factoring large numbers—a task that quantum computers can perform exponentially faster than classical ones. This has led to a race for developing quantum-resistant cryptographic algorithms. On the other hand, quantum cryptography, particularly quantum key distribution (QKD), offers new methods for secure communication based on the principles of quantum mechanics.
4. Quantum Machine Learning
Quantum machine learning leverages quantum computing’s ability to handle large, complex datasets more efficiently than classical computers. This could lead to breakthroughs in areas such as pattern recognition, natural language processing, and even autonomous systems. Although still in its infancy, quantum machine learning is being actively explored by companies like Google, Microsoft, and Xanadu, with the potential for substantial advancements in the coming years.
5. Financial Modeling
Financial markets involve complex systems with numerous variables, making them ripe for quantum computing applications. Quantum algorithms could enhance portfolio optimization, risk analysis, fraud detection, and option pricing. Several banks and financial institutions, such as JPMorgan Chase and Goldman Sachs, are already investing in quantum computing research to explore its potential in this domain.
The Road Ahead: When Will Quantum Computing Become Practical?
So, how close are we to seeing real-world applications of quantum computing? The answer depends on both technological advancements and the specific use cases being considered. For certain specialized applications—such as quantum cryptography and quantum key distribution—quantum technologies are already being deployed in limited, practical contexts. However, for more general-purpose quantum computing applications, we are likely still several years, if not decades, away.
Near-Term (0-5 Years)
In the near term, we are likely to see quantum computers solving highly specialized problems that are difficult for classical computers but not necessarily relevant for most commercial applications. This period will also see continued improvements in quantum hardware, error correction, and algorithm development. Pilot projects in industries like finance, logistics, and pharmaceuticals will explore the potential of quantum computing on a limited scale.
Medium-Term (5-15 Years)
Over the next decade, we may see the development of quantum computers with a few thousand qubits, capable of handling more complex calculations. Error correction will improve, making quantum computers more reliable for a broader range of applications. Industries such as materials science, drug discovery, and machine learning could see practical quantum applications emerge, leading to new discoveries and efficiencies.
Long-Term (15+ Years)
In the longer term, as quantum computers scale to millions of qubits and error rates are reduced to negligible levels, we may witness a quantum revolution across multiple sectors. At this stage, quantum computers could outperform classical computers in solving a wide range of complex problems, from cryptography and artificial intelligence to climate modeling and financial forecasting.
Conclusion
Quantum computing is rapidly advancing from a theoretical construct to a practical tool with the potential to transform multiple industries. While several challenges remain—particularly concerning error rates, scalability, and algorithm development—recent progress suggests that we are moving closer to real-world applications. For now, the technology is in a nascent stage, but as quantum hardware, software, and algorithms continue to mature, the next decade could see quantum computing emerge as a disruptive force in various fields, unlocking solutions to problems that were previously deemed unsolvable.
The journey toward practical quantum computing is still unfolding, and while the timeline remains uncertain, the destination promises to be transformative.
ALSO READ: Antitrust Actions Against Big Tech: Will They Make a Difference?